Breathe. Breathe. I repeated these words to myself like a mantra. At 18,400 feet, my body was craving oxygen, and I had to concentrate on pulling enough air into my lungs. I was on the summit of Cerro Toco, a stratovolcano overlooking Chile’s Chajnantor Plateau, now home to the Atacama Large Millimeter/submillimeter Array, one of the world’s premier radio telescopes. Between the thin atmosphere and the barren red terrain of the mountain, it felt like I was on Mars. My colleagues and I were testing the atmospheric conditions on Cerro Toco. If they were good enough, they might justify taking on the technical challenges of building an observatory at such a remote, high-altitude site.
Earth’s atmosphere is a problem for astronomers, and clouds frustrate many an observer. Atmospheric turbulence smears starlight, making stars appear to dance and flicker when close to the horizon. Molecules such as water vapor and carbon dioxide in the atmosphere absorb incoming starlight, particularly infrared light. With more than half of Earth’s air below the summit of Cerro Toco (a point repeatedly raised by my burning lungs), we hoped that new and exciting insights could come from a dedicated infrared telescope there.
The sense of adventure that had led me to this summit had also sparked my fascination with infrared astronomy, where scientists peer at the cosmos in light too red for the human eye to see. Infrared light tends to come from the dimmest and most distant objects observable. One class of objects best seen in the infrared is brown dwarfs. When I was in graduate school in the early 2000s, these bodies had only recently been discovered, and they presented many tempting mysteries. I came to be captivated by these uncanny orbs, which, in terms of their classification, occupy a boundary zone between stars and planets. I wondered where and how they formed and what they were like. I learned through my research that in addition to being interesting in their own right, brown dwarfs serve as an important bridge to our understanding of both planets and stars, with temperatures and masses intermediate between the two. Now I and other brown dwarf astronomers are enjoying a sweet spot for research—there are still many brown dwarfs waiting to be discovered, and we can build on the wealth of previous research to uncover new details of physical processes at work on these objects. We finally have the technological tools to study the atmospheres of brown dwarfs, for example, as well as their wind and rotation speeds, and to try to determine whether they might even host planets of their own.
On supporting science journalism
If you're enjoying this article, consider supporting our award-winning journalism by subscribing. By purchasing a subscription you are helping to ensure the future of impactful stories about the discoveries and ideas shaping our world today.
In-Between Objects
Most stars are powered by the fusion of hydrogen into helium, a wonderfully stable process that keeps stars burning at the same temperature and brightness for billions of years. But if a would-be star never reaches high-enough temperatures or pressures to sustain hydrogen fusion, it is a brown dwarf, with a maximum mass of 8 percent of our sun’s, or about 80 times the mass of Jupiter.
Recent studies indicate that brown dwarfs are nearly as common as stars, and they are everywhere. Brown dwarfs have been found in stellar nurseries alongside young protostars. They have been found in binary systems paired with white dwarfs, having survived potential engulfment by the white dwarf’s previous red giant form. (Our sun, a yellow dwarf star, will one day turn into a bloated red giant, and after it dies, it will become a white dwarf.) Some of the closest stellar systems to our sun are brown dwarfs—the third and fourth nearest extrasolar systems, at 6.5 and 7.3 light-years, respectively (the closest are Alpha Centauri and Barnard’s star). And yet, despite their ubiquity, most people have never heard of brown dwarfs.
Although they lack hydrogen fusion, brown dwarfs do emit light—thermal radiation from the heat within them. They start out relatively hot (around 5,000 degrees Fahrenheit), and over the subsequent billions of years, they cool and dim. Brown dwarfs never die; they spend eternity cooling off and fading away. The coldest known brown dwarf checks in at a temperature below the freezing point of water. Because they are so cool, most of the light they emit is at infrared wavelengths. They are far too faint for the unaided human eye to see in our night sky, but if we could look at them up close, they would probably have a dull orange-red or magenta hue.
In the more than two decades since astronomers began studying brown dwarfs, we have formed a fairly clear picture of their basic characteristics. Like our sun, brown dwarfs are composed almost entirely of hydrogen. The temperatures in their upper atmospheres are cool enough, however, that a variety of molecules can form. Signatures of water vapor are seen in nearly all brown dwarfs. As they cool further, their atmospheric chemistry changes, and different molecules and clouds become predominant. The evolution of a brown dwarf’s atmosphere depends on its mass and age. Imagine a brown dwarf with a mass 40 times that of Jupiter, for instance. For the first 100 million years, it will have an atmospheric composition similar to that of a red dwarf star, with titanium oxide and carbon monoxide present in the mix. Between 100 million and 500 million years, the atmosphere will cool, and dusty clouds made of minerals such as enstatite and quartz will form. Roughly a billion years after that, the clouds will break up and sink, and methane will become the dominant molecular species in the upper atmosphere. The coolest known brown dwarf shows evidence of water-ice clouds, as well as water vapor and methane. We expect its atmosphere to contain significant amounts of ammonia, similar to what we see on Jupiter.
Beyond these properties, however, there are many things about brown dwarfs that we do not yet know. The mysterious nature of these objects has inspired some far-fetched ideas. Brown dwarfs were once considered to be a possible reservoir of dark matter, although this idea was quickly abandoned when it became clear that brown dwarfs emit light (that is, they are not dark) and that their contribution to the total mass of our galaxy is small. More recently, scientists proposed that life could form in the cool upper regions of brown dwarfs’ atmospheres—an idea that brown dwarf experts quickly squashed because the dynamics are such that any life-form would cycle into deeper layers of the atmosphere that are hot and inhospitable.
And then there was the hoax of the Nibiru cataclysm, a prophesy put forward in 1995 that predicted an imminent, disastrous encounter between Earth and a brown dwarf. Astronomers would be very excited to see a brown dwarf up close, but there is no scientific evidence to support this doomsday scenario, and a brown dwarf would be visible for hundreds or thousands of years prior to any close encounter.
The First Brown Dwarfs
Scientists predicted brown dwarfs in the 1960s based on what they knew about how stars and planets form. It seemed that this intermediate category should exist, but astronomers were not finding any such objects in the sky. It turned out that brown dwarfs are simply very, very faint, and most of the light they emit is infrared. And infrared technology was still in its infancy—just not up to the task. Then came the year 1995, a big one for astronomy. Astronomers Michel Mayor and Didier Queloz found 51 Pegasi b, the first exoplanet known to be orbiting a regular star. Perhaps more important, at least to this highly biased author, the first brown dwarfs were discovered.
Teide 1 was identified in the famous Pleiades star cluster. Astronomers Rafael Rebolo López, María Rosa Zapatero-Osorio and Eduardo L. Martín first spotted it in optical images from the 0.80-meter telescope at the Teide Observatory in the Canary Islands. The object was young, still glowing slightly from its formation. The team observed the signatures of several elements in its atmosphere, including lithium.* Stars usually burn up lithium as soon as they form, so this amazing detection proved that nuclear fusion was not occurring. They published their finding in September 1995.
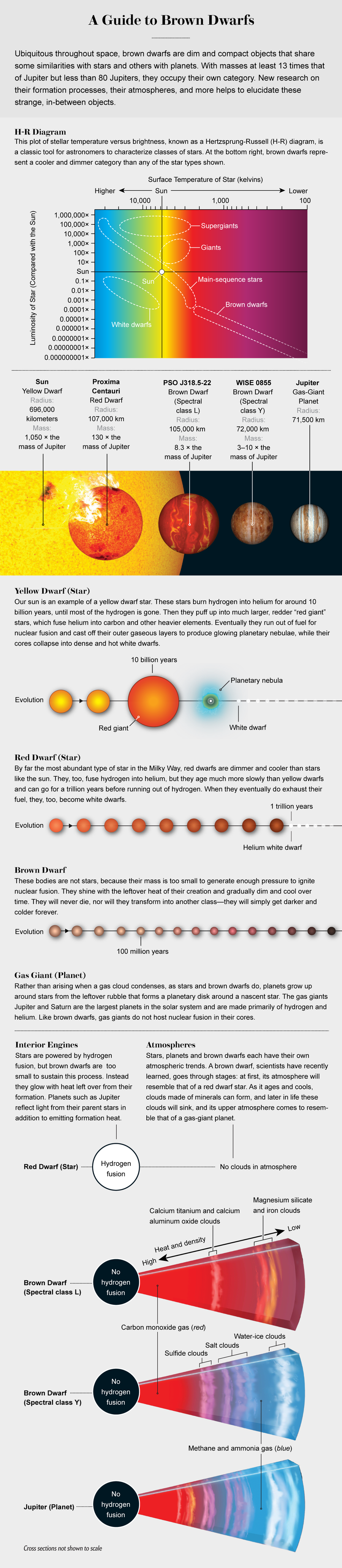
Credit: Illustration by Ron Miller (objects and atmospheres) and Jen Christiansen (H-R diagram)
Two months later astronomers announced the discovery of a second brown dwarf, Gliese 229B, a companion to another star. A group of astronomers at the California Institute of Technology and Johns Hopkins University first saw the object in an infrared image from the Palomar Observatory. They immediately knew that it was strange. It had unusual colors and displayed the signature of methane in its atmosphere. Conditions must be very cold for methane to be present because the highly reactive molecule usually turns into carbon monoxide at higher temperatures. Later observations revealed that the brown dwarf is about the same width as Jupiter, with a diameter of nearly 129,000 kilometers, but much denser, with 70 times as much mass.
By the time I started graduate school in 2000, we knew of more brown dwarfs, though not that many. I was focused on building infrared instruments, and I needed a subject for my research topic. My Ph.D. adviser studied star formation, so I decided to search for brown dwarfs in star-forming regions. I ended up discovering a good number of brown dwarfs in my thesis work, including some that were the first known to have masses putting them near the range of planets. At the time we had no idea how these things formed, and we did not know whether there was a lower-mass threshold, but we started finding smaller and smaller objects.
All in all, my thesis work published fewer than 20 new brown dwarf discoveries, but they made a significant contribution to the total number known. Since then, new instruments have found many, many more. The main contributors were the 2 Micron All Sky Survey (2MASS), an infrared survey conducted in the early 2000s, and the Wide-field Infrared Survey Explorer (WISE), a space telescope launched in 2009. The current tally of brown dwarfs is about 3,000. There are many more to be found, though—estimates suggest that the Milky Way contains between 25 billion and 100 billion brown dwarfs.
Formation Scenarios
As the lowest-mass outcome of the star-formation process, brown dwarfs offer astronomers a unique chance to deepen our understanding of the basic steps involved in the birth of stars and planets. Stars form in complexes of gas (mostly molecular hydrogen) and dust known as molecular clouds. If a molecular cloud contains enough mass, gravity can overcome the gas pressure supporting the cloud and cause it to collapse into a star. During the collapse, any small amount of rotation in the cloud becomes amplified, much like how ice skaters spin faster when they pull their arms in. This rotation of the cloud material leads to the formation of a circumstellar disk of matter surrounding the nascent star, which then becomes a crucible for planet formation.
When brown dwarfs were first discovered, astronomers assumed they might form in a process similar to that for stars, but they were perplexed as to how the gravity from such a small mass was able to overcome gas pressure and initiate a collapse. In writing this article, I looked back over some grant and telescope proposals from early in my career, most of which were aimed at better understanding the formation mechanism of brown dwarfs. At the time there were several competing ideas. Some theories involved disrupting the formation of a star before it had reached its final mass. Perhaps some process physically removed the brown dwarf or burned off its natal environment, leaving behind a miniature star?
Other hypotheses invoked a scaled-down version of star formation or a scaled-up version of planet formation. This is a lovely example of using a variety of possible theories to make distinct, testable predictions. As we discovered the ubiquity of circumstellar disks around brown dwarfs, determined the distribution of stellar and brown dwarf masses in a variety of environments, and mapped the orbits of brown dwarfs in binary pairs, it became clear that most brown dwarfs seem to form like scaled-down stars—but from a smaller reservoir of gas. And the fact that brown dwarfs form circumstellar disks raises the tantalizing possibility that they host planets. Although we have never seen any for sure, it is very likely that planets grow in these disks just as they do around stars. Scientists hope the coming years will finally see the confirmed discovery of worlds orbiting brown dwarfs.
Recently researchers discovered isolated brown dwarfs with masses similar to those of giant planets (less than 13 times the mass of Jupiter), which again raised the question of how they might have formed. Could some of these planetary-mass brown dwarfs have arisen in the circumstellar disks of more massive stars—in other words, formed just as planets do?
To test the mechanism for the formation of planetlike masses, my colleagues and I proposed a survey with the Hubble Space Telescope. Because Hubble is in orbit, it avoids the smearing and absorption of light by Earth’s atmosphere, which makes it ideal for imaging binary pairs of brown dwarfs. Through this survey, in 2020 we discovered a unique system of brown dwarfs that strongly supports a starlike-formation mechanism for planetlike masses. The system, Oph 98 AB, is very young in cosmic terms (three million years old), and its two components weigh in at 15 and eight times the mass of Jupiter. These extremely low-mass objects are separated by 200 times the distance between Earth and the sun. Because Oph 98 A and B are so light and so widely separated, the system has the lowest gravitational binding energy of any known binary pair. The weak binding energy means that these bodies must have formed in their current orientation, rather than originating elsewhere and later becoming a pair, which points to a starlike-formation mechanism. And the young age of the system (yes, we consider three million years young!) means that planetary-mass objects apparently do not take any longer to form than stars.
New Insights
Brown dwarf science has now reached a stage where we are able to make more precise measurements and ask more detailed questions than ever before about these still mysterious objects. Among the most interesting recent discoveries are the coldest brown dwarfs, known as Y dwarfs. These objects have temperatures ranging from 350 degrees F down to –10 degrees F. I love to joke when working on Y dwarfs that I am studying the coolest systems in the galaxy! Though not quite as cold as Jupiter (–234 degrees F), these Y dwarfs have enabled us to make the first meaningful comparison between brown dwarfs and the atmospheres of the giant planets in our solar system. Y dwarfs are difficult to observe because they are both cool and very dim. The light they do emit is predominantly in the infrared range, at wavelengths of three to five microns, where Earth’s atmosphere makes observations difficult.
Regardless, my colleagues and I have published spectra of several Y dwarfs and used theoretical models to infer the presence of water-ice clouds, as well as a significant amount of vertical mixing in the atmosphere. In this same wavelength range, Jupiter emits its own light (rather than just reflecting the light of our sun) and shows significant vertical mixing as well. Our hope is that by studying Y dwarfs, we will be able to disentangle properties of Jupiter that come from its planetary nature—in other words, the fact that it formed in the circumstellar disk of our sun and is constantly illuminated by sunlight—and properties that may be ubiquitous among cool gaseous objects, be they planets, exoplanets or brown dwarfs. Thus far our studies are showing that highly dynamic atmospheres tend to be the norm.
These insights about brown dwarf atmospheres have led to a new subfield: exometeorology. Although brown dwarfs are too far away for us to visually examine their atmospheric features, we can see their imprint through changes in brightness. As a cloud or other feature rotates in and out of view, it changes the light coming from the brown dwarf. Astronomers have analyzed the brightness variations of brown dwarfs over many rotations and have created maps of their spots and bands, which look remarkably like the familiar stripes and storms on the giant planets in our own solar system. Some brown dwarfs have been found to change in brightness by up to 25 percent over one rotation. The results of these studies are leading us to better understand atmospheric processes more generally—we have found that brown dwarfs with temperatures at which clouds break up show large variations in brightness and that young objects tend to show greater variability in brightness.
Scientists have also discovered other similarities between brown dwarfs and gas giants. Both, for example, tend to have strong magnetic fields and aurorae, as revealed by radio observations of the signatures of charged particles spiraling in their magnetic fields. The measured magnetic field strengths for brown dwarfs are 1,000 times stronger than Jupiter’s magnetic field and 10,000 times stronger than Earth’s. I like to imagine what the night sky might look like from one of these brown dwarfs—given the beauty of Earth’s aurora borealis, it would likely be a spectacular sight.
Recently a student’s question prompted another project to examine how the atmospheres on brown dwarfs compare with those on planets. When I teach courses in introductory astronomy, we cover the planets of the solar system (and of course, I sprinkle in a lot of information about brown dwarfs as well). A tidbit I present is that the length of a Jovian day depends on how you measure it. If you clock the motion of visible features in Jupiter’s equatorial region, you measure a rotation period that is five minutes shorter than the rotation period measured in the radio signal, which probes its interior rotation. A student asked me why this difference in rotation period occurs, and I replied that it was because Jupiter’s equatorial features are pushed along by strong zonal winds. The winds on Earth are driven by the redistribution of solar energy, but we are not sure to what degree this applies to Jupiter’s winds.
After the lecture, I started thinking about this further. Astronomers have measured radio emission in brown dwarfs, which occurs via the same mechanism as Jupiter’s radio emission, so we can measure an interior rotation period. And we can use our method of monitoring brightness changes to measure the atmosphere’s rotation period. Thus, I hatched an idea to measure the wind speed on a brown dwarf for the first time. The best candidate we had to try out the technique was a methane brown dwarf with confirmed radio emission. To determine the wind speed, we would need to measure both periods to a precision of less than 30 seconds. My colleagues and I submitted a proposal to use the Spitzer Space Telescope to measure the brown dwarf’s brightness variations and applied to use the Karl G. Jansky Very Large Array in New Mexico to measure a more precise radio period. It still feels like a small miracle that our measurements revealed a period difference of just more than a minute, which equates to a wind speed of 2,300 kilometers per hour. We published our findings last year in the journal Science. This high wind speed on an isolated brown dwarf means that atmospheric winds are not always driven by the redistribution of solar energy, leaving open the question of whether Jupiter’s winds are driven by the sun.
Astronomers continue to search for more brown dwarfs. Some surveys focus on identifying large samples of brown dwarfs via deep imaging surveys of the whole sky such as 2MASS, WISE, and the Panoramic Survey Telescope and Rapid Response System (Pan-STARRS). Citizen scientists have also become involved in the search through projects such as Backyard Worlds, which allows anyone to examine WISE data for signs of brown dwarfs and other moving objects. We expect that upcoming large surveys with the Vera C. Rubin Observatory (due to begin observing early next year) and the Nancy Grace Roman Space Telescope (launching in 2025) will work to further complete our census of brown dwarfs.
Sadly, we could not get funding for the telescope on Cerro Toco, and it was never built. But once the James Webb Space Telescope is launched later this year, astronomers will have an unprecedented look at brown dwarfs in the infrared, without interference from Earth’s atmosphere. The first cycle of observations planned includes programs to study the atmospheric chemistry of Y dwarfs and the cloud composition of dusty brown dwarfs and even a search for planetary systems around brown dwarfs. Exciting times are certainly ahead for those of us who study some of the cosmos’s most overlooked objects.
*Editor’ Note (10/18/21): This sentence was edited after posting to correct the description of the observation of lithium and other elements in Teide 1’s atmosphere.