In a part of Los Alamos National Laboratory (LANL) that some call the Mesa, a beam of protons shoots down a mile-long tunnel through the New Mexico landscape. The particles are moving so fast that if they weren’t confined within the structure, they could circle the Earth 2.5 times in a second.
Five different facilities at the laboratory “sip” from this beam, pulling the protons they need for various experiments. One of these, called the Isotope Production Facility, smashes the protons into a stationary target. The protons embed themselves in the target’s atoms, transmuting them into new elements.
The Isotope Production Facility is currently involved in a positive (pun intended) effort for the medical industry: producing a substance called actinium 225, an isotope with 89 protons and 136 neutrons. Isotopes are different forms of the same element that have the same number of protons but different numbers of neutrons; the most common actinium isotope is 227, with two more neutrons than 225. Isotopes are radioactive when their nuclei are unstable, with a twitchy combination of protons and neutrons, and they rid themselves of excess energy by emitting alpha, beta or gamma rays. Actinium 225’s particular radioactivity is potentially potent in the fight against prostate cancer, and studies on its effectiveness against other malignancies are in the works. Although many radioactive isotopes exist, actinium 225’s emissions are strong enough to damage cancer cells without doing as much harm to healthy ones. And this isotope dissipates in a just-right amount of time, doing its job and then decaying away.
On supporting science journalism
If you're enjoying this article, consider supporting our award-winning journalism by subscribing. By purchasing a subscription you are helping to ensure the future of impactful stories about the discoveries and ideas shaping our world today.
For years, scientists have produced this species of actinium by waiting for thorium (element 90, with one extra proton than actinium) to decay. But that method is slow and doesn’t yield much at a time. If cancer treatments using actinium 225, currently in clinical trials, are approved by the U.S. Food and Drug Administration, the old way of doing things likely won’t meet demand.
Anticipating the potential need for actinium production to jump to a new energy level, the Department of Energy created a program in 2015 to develop new, bigger-batch production methods—uniting LANL, Oak Ridge National Laboratory (ORNL) in Tennessee and Brookhaven National Laboratory in New York State in the effort. Since then the labs have been working steadily on the project, hoping to be ready when the medical industry is.
As actinium 225 decays, it emits radiation in the form of alpha particles (two protons and two neutrons bound together). Kirk Rector, a program director at LANL and the lab’s point of contact for the Department of Energy’s Isotope Program, calls these particles “wrecking balls.” The energy they create is strong enough to break strands of DNA like a lightsaber slashing through two jump ropes engaged in a game of double Dutch.
But alpha particles are heavy (at least for particles), so they don’t travel very far—just as far as a few cells lined membrane to membrane—meaning doctors can target radiation therapy to the right spot and limit damage to the surrounding tissue. Plus, actinium 225 has a half-life of just under 10 days, so when it’s placed in the body, it has enoughtime to reach the right cells but not so much time that it gets concentrated there, spewing too much radioactivity for too long.
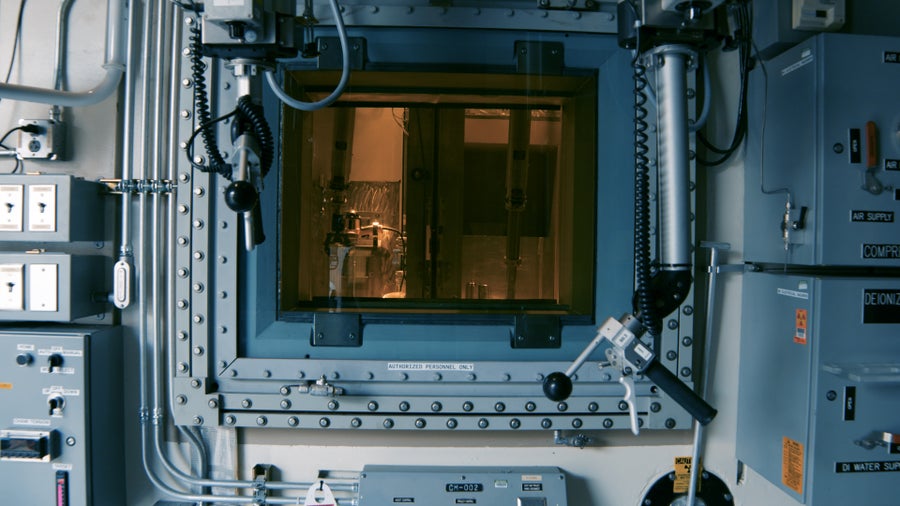
The Hot Cell at the Isotope Production Facility at the Los Alamos Neutron Science Center (LANSCE) is used for loading and unloading targets into a beam of protons for irradiation. Credit: Courtesy of Los Alamos National Laboratory
To help the actinium do no harm (or as little as possible), though, it needs an additional ingredient: something that leads it to seek out cancer cells and not healthy ones. Recently, researchers have developed FDA-approved treatments that can home in on a molecule on the surface of prostate cancer cells and deliver radiation to the problem area.
“When it recognizes a prostate cancer cell, it sticks to it,” Rector says. “And it holds on just to those cells.” Now similar treatments are being developed with actinium 225 along for the ride. Such targeted drugs could take that isotope’s radioactivity right where it’s supposedto inflict damage—like a “heat-seeking missile,” says Mitch Ferren, former associate director of business operations at the National Isotope Development Center. It’s one form of a kind of treatment called “targeted alpha” therapy.
Around a dozen clinical trials have involved or currently involve actinium 225, trying out treatments not just for prostate cancer but also for conditions such as leukemia, solid tumors and carcinomas. The initial development of a targeted-alpha compound involving actinium 225, back in 2013, “not only provided a new treatment option for prostate cancer but also demonstrated the potential of the technology for treatment of cancer in general,” says Alfred Morgenstern of the European Commission’s Joint Research Center, one of only a few places that currently produces actinium 225.
Studies so far seem promising—and that promise has a long historical basis. Researchers have known that actinium 225 would likely be useful for cancer treatment for more than 30 years, beginning with the publication of a paper entitled “The Feasibility of 255Ac as a Source of α-Particles in Radioimmunotherapy” in Nuclear Medicine Communications in 1993. Decades of research followed, but it was only after the compound for targeted treatment was synthesized that practical developments truly ramped up. Seeing that, the DOE stepped in to help increase the supply to meet future needs. “The demand has risen significantly, and it is extremely important that new production facilities come into operation soon to mitigate the supply shortage,” Morgenstern says.
Right now labs in Germany, Russia and Canada make this isotope, but most of Earth’s actinium 225 drips out of a supply of thorium 229 in the form of old nuclear waste that lives at ORNL. As this thorium ages, it decays into an isotope of radium, which then decays naturally into actinium 225. Weirdly, everyone calls this “milking the thorium cow.” But the cow’s flow is low, and there’s not enough milk for the trials, let alone the future treatments.
That’s why the DOE initiated what it calls the Tri-Lab Effort (because of the triple team of LANL, ORNL and Brookhaven) to build a more golden cow, producing actinium 225 in new ways.
“They did begin investigating various different alternative pathways,” says Karen Sikes, director of the National Isotope Development Center. But one stood out: shooting protons at targets made of thorium 232. Those protons smashing and staying put creates the right kind of actinium through a process called spallation. The Tri-Lab Effort has worked on the specific infrastructure and techniques to do so, using existing particle accelerators to create the shooting proton beams. It’s worked, with the first batches processed in 2018. In 2022, the Tri-Lab researchers were able to produce more than six times the amount they did in 2018, although that’s still likely a fraction of what the medical industry might eventually call for. At LANL, early work has begun on a new facility dedicated to producing isotopes like actinium 225. “This would allow us to dramatically scale up how much we can make,” Rector says.
“What we're doing right now is modern-day alchemy,” he proclaims. It’s a tad grandiose, but he’s not wrong: the fundamental idea of alchemy is taking an element that’s plentiful and cheap and transmuting it into something else that’s more rare and valuable. In the old days, that meant pseudoscientific efforts to shift lead into gold using something often called a philosopher’s stone, which purportedly held within its rockiness the forces of the universe.
Today that sounds silly. But, Rector says, “we actually think they were right. We can do those transformations. They just had the wrong magic rock.” The modern magic rock is a particle accelerator, which changes atoms’ identities using fast-paced protons. “We're harnessing the forces of the universe in order to transform one element into another,” Rector says.
The actinium 225 made using this Tri-Lab method does have a potential drawback, though, compared with the thorium-cow-made version: it’s mixed up with actinium 227 (which has two more neutrons), which can’t be easily separated from the desired isotope. “The advantage of the spallation process is that the target material thorium 232 is abundantly available, and production can theoretically be increased more easily,” Morgenstern says. “However, the process requires costly, large accelerators. And the product actinium 225 is contaminated with long-lived actinium 227, which causes issues with handling and waste disposal.”
Understanding if and how that impurity affects the supply may require different trials. But that’s already in motion, too: the FDA accepted a “drug master file” that described the facilities and production processes for accelerator-based actinium back in 2020.
When researchers or pharmaceutical companies want actinium 225 for their drugs, their orders may go through the National Isotope Development Center, which is a commercial arm of the DOE.
Some customers, such as actinium 225 shoppers, are in the medical industry, but other isotopes go in atomic clocks, spacecraft, computers and oil-and-gas instruments. Rather than competing with the private sector, the center only sells isotopes that the commercial industry doesn’t produce in adequate quantities or that have a production problem that calls for filling a gap. “What's certainly been a big effort over the last couple of years is reducing our dependency on foreign supply, in particular Russia and Ukraine,” Rector says, “and making sure that the isotopes that we need that are critical for industries such as semiconductor manufacturing or quantum computing are things that we have available within the United States.” Rector makes sure the right amount of the right isotopes are ready when they need to be, which is something the alchemists of old couldn’t manage.